Sensor System
Lorem ipsum dolor sit amet, quis nostrud exercitation ullamco laboris nisi ut aliquip ex ea commodo consequat.
Technology
Nanoplasmonic Sensing
Localized Surface Plasmon Resonance (LSPR)
Surface plasmons are collective oscillations of the free electron gas at the surface of metals that can be induced by an external electric field, including electromagnetic radiation (Figure 1). When the size of the metal structures is reduced to nanoscale dimensions, the electron oscillations are confined in the nanostructures creating a phenomenon known as localized surface plasmon resonance (LSPR). The excitation of LSRP gives rise to a strong enhancement of electromagnetic fields in the near-field region and the ability to concentrate electromagnetic radiation into particles of nanoscale dimensions has made plasmonic materials attractive for a large variety of applications, including molecular sensing.
For most types of silver and gold nanostructures, the resonance frequency is in the visible region of the electromagnetic spectrum, which gives rise to distinct colors for nanoparticle ensembles. The colors emanates from the extensive absorption and scattering (extinction) of light by the nanoparticles at the LSPR frequency. The resonance conditions are highly dependent on a number of parameters, including i) the nature of the nanostructures i.e. their size, shape, and composition ii) their interaction with other, closely spaced metal nanostructures and iii) the dielectric properties of the surrounding medium.
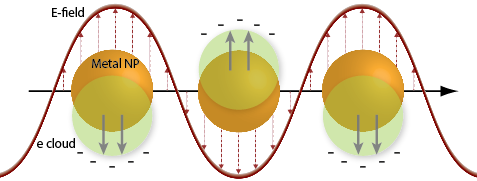
Figure 1: Schematic illustration of localized surface plasmon resonance in spherical gold nanoparticles induced by an external electric field.
Nanoplasmonic Sensing
Since the LSPR frequency is sensitive to changes around the metal nanostructures, they can act as sensitive probes for detecting changes in the refractive index (RI) in their close proximity caused by for instance the adsorption of biomolecules to the metal surface. The RI dependency of the LSPR is the basis of nanoplasmonic sensing. The highly localized sensing volume around metal nanostructures makes these materials attractive signal transducers in miniaturized sensors with high sensitivity to local RI changes.
Nanoplasmonic sensing is an optical technique that enables robust label-free detection of biomolecular interactions in real-time. Small changes in the refractive index caused by binding of biomolecules to the metal surface alter the LSPR frequency which can be detected spectrophotometrically (Figure 2). Nanoplasmonic sensing is a highly generic technique where specificity is attained by attaching capturing molecules (ligands) to the surface of the nanoparticles. The sensor system can thus be addressed towards various (bio)molecular analytes by using the appropriate ligands.
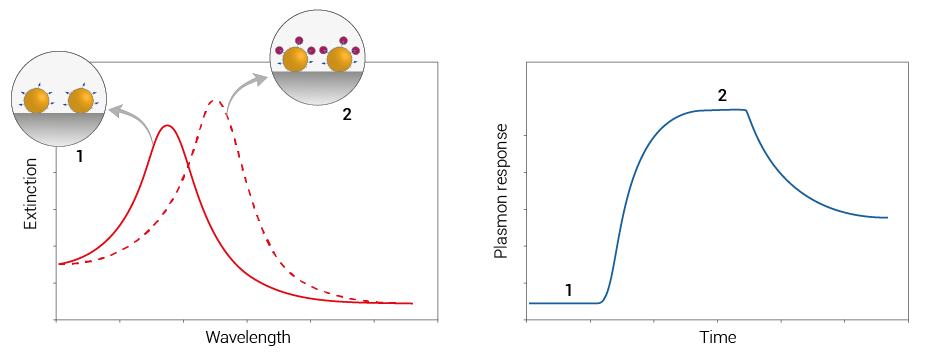
Figure 2: Schematic illustration of the basic concept behind nanoplasmonic sensing using plasmonic nanoparticles. Molecular interactions that take place at the surface of a metal nanoparticle change the conditions for the LSPR excitation that result in measurable changes in the extinction spectra, which enables real-time, and label-free detection of the binding event.
Nanoplasmonic sensing has several advantages
-
- Label-free, real-time detection
- Flexible configurations and miniaturization possible
- High sensitivity (from small peptides to bacteria)
- Surface sensitive detection with limited bulk contribution
- No active temperature control needed